Nassrene Y. Elmadhun, Ashraaf A. Sabe, Antonio D. Lassaletta, Louis. M. Chuand Frank W. Sellke*
Division of Cardiothoracic Surgery, Cardiovascular Research Center, Warren Alpert School of Medicine, Brown University, Providence, RI
*Address for Correspondence: Frank W. Sellke, Division of Cardiothoracic Surgery, Cardiovascular Research Center, Warren Alpert Medical School of Brown University, 2 Dudley Street, MOC 360, Providence
Dates: Submitted: 07 December 2016; Approved: 07 February 2017; Published: 15 February 2017
Citation this article: Elmadhun NY, Sabe AA, Lassaletta AD, LM AD. Alcohol Alters the Insulin Signaling Pathway in Ischemic Myocardium. J Res Diabetes Metab. 2017;3(1): 001-007
Copyright: © 2017 Elmadhun NY, et al. This is an open access article distributed under the Creative Commons Attribution License, which permits unrestricted use, distribution, and reproduction in any medium, provided the original work is properly cited.
Keywords: Insulin signaling; Alcohol; Ischemia; Myocardium; Metabolic syndrome
Abstract
Background: In a previous study, we demonstrated that swine supplemented with alcohol had improved insulin signaling in the heart. However, the effect of alcohol on insulin signaling in the setting of metabolic syndrome in the heart remains unknown. We developed a follow-up study to evaluate the effects of alcohol on ischemic myocardium in animals with metabolic syndrome.
Materials and Methods:26 Yorkshire swine were fed a hypercaloric diet for 4 weeks, followed the placement of an ameroid constrictor to induce chronic myocardial ischemia. Postoperatively, the animals were split into three groups: hypercholesterolemic diet alone (n = 9), the hypercholesterolemic diet with vodka (n = 9), and hypercholesterolemic diet with wine (n = 8) for 7 weeks. Animals underwent intravenous dextrose challenge prior to euthanasia and tissue collection.
Results:Alcohol supplementation decreased glucose tolerance compared to the control. Alcohol also decreased expression of pro-insulin signaling proteins AKT, pAKT, AMPK, pMTOR and increased expression of GSK3 , which decreases insulin sensitivity. There was also increased GLUT1 and GLUT4 immunostaining in the myocardium of animals supplemented with alcohol compared to the control.
Conclusion:Both HCVOD and HCW groups demonstrate glucose intolerance and aberrant insulin signaling with increased expression of pro-insulin signaling proteins down-regulation of insulin signaling activators. The mixed signals may result in the observed insulin insensitivity. Moderate alcohol consumption seems to have a complex and negative effect on insulin signaling in ischemic myocardium.
Abbreviations
HCC: Hypercholesterolemic Diet Control; HCVOD: Hypercholesterolemic Diet+Vodka ; HCW: Hypercholesterolemic Diet+Wine ; IRS: Insulin Receptor Substrate; pIRS: phosphorylated Insulin Receptor Substrate; PI3K: Phosphoinositide 3-Kinase; AKT: Protein Kinase B; pAKT: Phophorylated Protein Kinase B; GSK-3: Glucose Synthase Kinase-3; pGSK-3: Phosphorylated Glucose Synthase Kinase-3; GS: Glucose Synthase; MTOR: Mammalian Target of Rapamycin; pMTOR: Phosphorylated Mammalian Target of Rapamycin; AMPK: AMP-Activated Protein Kinase; pAMPK: phosphorylated AMP-Activated Protein Kinase; GLUT1: Glucose Transporter Type 1; GLUT4: Glucose Transporter Type 4; PAS: Periodic Acid Schiff
Introduction
It is estimated that between the year 2000 and 2030, there will be a 37% increase in the prevalence of type 2 diabetes in worldwide [1]. Paralleling the epidemic rise in diabetes, metabolic syndrome and its component atherogenic risk factors, including hypertension, dyslipidemia, obesity, and insulin resistance are also rising at an alarming rate [2]. Cardiovascular disease remains the leading cause of morbidity and mortality in diabetic patients [3]. Despite advancements in the management of acute myocardial infarction, diabetic patients have double the mortality compared to non-diabetics, and diabetics with no previous history of myocardial infarction have a higher re-infarction risk compared to non-diabetics who have a history of infarction [4,5]. For patients undergoing coronary artery bypass grafting, hyperglycemia and insulin resistance is associated with adverse clinical outcomes, and aggressive glucose control has been shown to improve morbidity and mortality in both diabetic and non-diabetic patients [6].
Epidemiologic studies have shown that alcohol consumption can modify risk factors for developing cardiovascular disease. Excessive and chronic alcohol consumption (>60g ethanol daily) increases the risk of type 2 diabetes, whereas low-moderate alcohol consumption (15-30g ethanol daily) improves insulin sensitivity, and reduces the risk of type 2 diabetes [7-10]. The J-shaped relationship between alcohol and insulin sensitivity also applies to overall morbidity, mortality, and cardiovascular disease risk [11].
We previously demonstrated that the heart-healthy polyphenol in red wine, resveratrol, improved glucose tolerance and insulin signaling in the liver and skeletal muscle [12,13], but did not significantly change insulin signaling in the myocardium in a swine model of metabolic syndrome and chronic ischemia [14]. In another study, we reported that moderate consumption of unaltered, pharmaceutical grade ethanol (45g ethanol daily) improved insulin signaling despite normoglycemia in a swine model of chronic myocardial ischemia and normal BMI [15]. Given these results, we sought to determine the effect of moderate doses of resveratrol-containing alcohol (red wine), and resveratrol-free alcohol (vodka) on myocardial insulin signaling in a clinically relevant swine model of metabolic syndrome and chronic ischemia.
Materials and Methods
Animal model
Twenty-six male Yorkshire mini swine (Parsons Research, Amherst, MA) were fed 500g/ day of a high-cholesterol diet consisting of 4% cholesterol, 17.2% coconut oil, 2.3% corn oil, 1.5% sodium cholate, and 75% regular chow (Sinclair Research, Columbia, MO). After 4 weeks of a high-cholesterol diet, all animals underwent ameroid constrictor placement to the left circumflex artery to induce chronic cardiac ischemia. Postoperatively, the animals were split into 3 different groups according to diet supplementation for an additional 7 weeks. The control group was continued on a hypercholesterolemic diet alone (HCC n = 9). The hypercholesterolemic vodka (HCVOD n = 9) and hypercholesterolemic wine (HCW n = 8) groups were supplemented with 112 mL of vodka (Rubinoff Vodka, Somerville, MA) (40% EtOH/V) and 375 mL of red wine daily (2009 Pinot Noir, Black Mountain Vineyard, Napa and Sonoma, CA) (12.5% EtOH/V) respectively. Resveratrol content of the red wine was 0.3-0.5 g/ mL and was quantified by laser chromatography-mass spectroscopy [16]. After 7 weeks of diet supplementation, all animals underwent euthanasia and cardiac tissue harvest. All animals were observed to ensure complete consumption of food and supplement, had unlimited access to water and were housed in a warm non-stressful environment for the duration of the experiment. Only male animals were studied in this model to eliminate the variability that sex hormones may have on insulin signaling.
Surgical interventions
Anesthesia:Anesthesia was induced with an intramuscular injection of telazol (4.4 mg/ kg). Animals were endotracheally intubated, mechanically ventilated at 12-20 breaths per minute, and general anesthesia was maintained with a gas mixture of oxygen at 1.5-2 liters/ min and isoflurane at 0.75-3.0% concentration.
Ameroid constrictor placement: Animals were given a single dose of antibiotic prophylaxis, intravenous enrofloxacin 5 mg/ kg, and general anesthesia was induced and maintained. Animals were prepped and draped in the usual sterile fashion. The heart was exposed through a left mini-thoracotomy. The left atrial appendage was retracted and the proximal left circumflex artery was dissected at the take-off of the left main coronary artery. The ameroid constrictor was placed around the left circumflex artery (Research Instruments NW, Escondido, CA) approximately 1cm distal to the takeoff from the left main coronary artery. The pericardium was loosely re-approximated followed by a layered closure of the surgical incision. Post-operative pain was controlled with a single dose of intramuscular Buprenorphine (0.03 mg/ kg) and 72 hour Fentanyl patch (4 g/ kg). All animals received 325 mg of aspirin daily for thromboembolic prophylaxis starting 1 day pre-operatively and continuing for a total of 5 days. All animals continued perioperative antibiotics: enrofloxacin 68 mg orally daily for 5 days.
Cardiac harvest: Under general anesthesia, the heart was exposed through a median sternotomy and animals were euthanized by exsanguination. Cardiac tissue from the ischemic territory in the left circumflex artery distribution was collected for further analysis.
The Institutional Animal Care and Use Committee of the Rhode Island Hospital approved all experiments. Animals were cared for in compliance with the "Principles of Laboratory Animal Care" formulated by the National Society for Medical Research and the "Guide for the Care and Use of Laboratory Animals" (NIH publication no. 5377-3 1996).
Serologic studies
Blood samples were drawn from the jugular vein and serum insulin levels were quantified by the chemistry laboratory at the Rhode Island Hospital, Providence RI at the time of harvest. Blood glucose measurements were taken at baseline (fasting) and 30 and 60 minutes after an intravenous 0.5 mg/ kg dextrose infusion at the time of ameroid constrictor placement (before animals were supplemented with alcohol), and again at the time of harvest (after the HCVOD and HCW animals were supplemented with vodka and wine respectively for 7 weeks).
Protein expression western blot
Frozen tissue samples were homogenized and in a radio-immunoprecipitation assay solution (Boston BioProducts, Ashland, MA) supplemented with protease and phosphatase inhibitors. Forty micrograms of whole-tissue lysates were fractionated by sodium dodecyl sulfate-polyacrylamide gel electrophoresis 4-12% Bis-Tris gels (NuPage Novex Mini Gel, Invitrogen, Carlsbad, CA) for targets <100 kilodaltons or were fractionated by sodium dodecyl sulfate polyacrylamide gel electrophoresis 3-8% Tris-Acetate gel (NuPage Novex Mini Gel, Invitrogen) for targets >100 kilodaltons. Gels were subsequently transferred to polyvinylidene difluoride membranes (Millipore, Bedford, MA). Membranes were incubated overnight at 4 with primary antibodies at dilutions recommended by the manufacturer against phosphorylated insulin receptor substrate 1 (pIRS1 Ser612), IRS1, IRS2, phosphoinositide 3-kinase (PI3K), phosphorylated protein kinase B (pAKT Thr 308), pAKT (Ser 473), AKT, glucose synthase kinase 3 (GSK3), phosphorylated AMP-activated protein kinase (pAMPK Thr 172), AMPK, phosphorylated mammalian target of rapamycin (pMTOR S2481), MTOR (all from Cell Signaling, Danvers, MA), and phosphorylated IRS2 (Ser 731) (Abcam Cambridge, MA). Membranes were incubated with the appropriate horseradish peroxidase-linked secondary antibody for one hour at room temperature (Jackson ImmunoResearch, West Grove, PA). Immune complexes were visualized with enhanced chemiluminescence and images were captured with a digital camera system (G-Box, Syngene, and Cambridge, England). Band densitometry was quantified as arbitrary light units using Image-J software (National Institutes of Health, Bethesda, MD). All membranes were probed with GAPDH to correct for loading error.
GLUT1 and GLUT4 immunohistochemical staining
Frozen myocardial tissue samples were sectioned (12- m-thickness) and fixed in 10% formalin for 10 minutes. Sections were blocked with 1% bovine serum albumin in phosphate buffered saline for 1 hour at room temperature and incubated with anti-GLUT1 (Abcam) or anti-GLUT4 (Epitomics, Burlingame, CA) antibody overnight at 4 . Sections were then incubated with the DyLight conjugated anti-rabbit antibody (Jackson ImmunoResearch) for 45 minutes, and then mounted with Vectashield with 4',6-diamidino-2-phenylindole (Vector Laboratories, Burlingame, CA). Three random images from each tissue sample were captured at X20 magnification with a Nikon E800 Eclipse microscope (Nikon, Tokyo, Japan) at the same exposure and signal intensity was analyzed using Image J software.
Oil Red O and periodic Acid-Schiff histologic staining
Oil Red O and Periodic Acid-Schiff and staining were performed on frozen tissue sections by the Pathology and Histology core facility at Rhode Island Hospital. Images were captured at X20 magnification with a Nikon E800 Eclipse microscope (Nikon) from each representative tissue section in a random fashion.
Data analysis
All results are reported as a mean standard error of the mean. A two-way ANOVA was used to compare the means between groups of the dextrose challenge data followed by a post-hoc Bonferroni test using GraphPad Prism 5.0 Software (GraphPad Software Inc., San Diego, CA). A one-way ANOVA was used to compare means between groups followed by a post-hoc Bonferroni test using GraphPad Prism 5.0 Software for all other studies.
Results
Animal model
All animals that were included in the analysis survived the entire experiment. We previously reported that there was no difference in body mass index in the HCC, HCVOD and HCW groups at the time of harvest [17]. At the time of ameroid placement surgery, before animals started alcohol supplementation diets, we also found that there was no difference in the baseline blood glucose or in blood glucose after 30 and 60 minutes after dextrose challenge in the HCC, HCW and HCVOD groups. After 7 weeks of alcohol supplementation, all animals had similar baseline blood glucose, but at 30 and 60 minutes after dextrose infusion, the HCVOD and HCW groups had significantly elevated blood glucose compared to HCC (Table 1). We also reported that the alcohol groups also had elevated fasting insulin levels compared to HCC, although only the HCW group was significantly elevated [17].
Table 1
Table 1: Serum Glucose after Dextrose Challenge. | ||||
A. Dextrose Challenge After 4 Weeks High Fat Diet | ||||
0 min | 30 min | 60 min | ANOVA p Value | |
HCC | 76.2 7.8 | 196.0 15.3 | 141.3 16.3 | 0.55 |
HCW | 72.6 5.3 | 168.1 20.5 | 111.0 15.7 | |
HCVOD | 69.6 7.1 | 177.8 11.1 | 105.9 12.6 | |
B.Dextrose Challenge After 7 Weeks Alcohol Supplementation | ||||
0 min | 30 min | 60 min | ANOVA p Value | |
HCC | 62.3 4.9 | 173.7 4.9 | 102.0 8.0 | 0.02 |
HCW | 67.1 7.2 | 215.6 7.5 * | 152.4 8.5* | |
HCVOD | 57.3 4.7 | 201.8 8.7 * | 120.4 12.8* | |
Animal blood glucose (mg/ dL) after 4 weeks of a high fat diet alone (A) and after 7 weeks of diet with alcohol supplementation (B). * Indicates p < 0.05 compared to HCC. HCC: High Cholesterol Control. HCW: High Cholesterol + Wine. HCVOD: High Cholesterol + Vodka. |
Table 2
Table 2:Insulin signaling protein expression in chronically ischemic myocardium | |||||
HCC | HCVOD | HCW | ANOVA p | ||
IRS1 | 1.000.15 | 1.000.06 | 0.790.06 | 0.25 | |
pIRS1 (Ser 612) | 1.000.18 | 0.920.06 | 0.940.09 | 0.89 | |
IRS2 | 1.000.17 | 0.810.05 | 0.660.05 | 0.09 | |
pIRS2 (Ser 731) | 1.000.09 | 0.900.08 | 1.210.08 | 0.1 | |
PI3K | 1.000.10 | 1.460.13 | 1.160.08 | 0.02 | T |
AKT | 1.000.11 | 0.470.07 | 0.550.09 | 0.001 | T* |
pAKT (Thr 308) | 1.000.11 | 0.740.06 | 0.620.08 | 0.02 | * |
pAKT (Ser 473) | 1.000.15 | 0.760.09 | 0.590.10 | 0.01 | * |
AMPK | 1.000.13 | 0.560.03 | 1.010.20 | 0.05 | T |
pAMPK (Thr 172) | 1.000.09 | 1.100.08 | 1.110.08 | 0.61 | |
GSK3 | 1.000.11 | 1.410.13 | 1.400.14 | 0.05 | T* |
MTOR | 1.000.09 | 1.030.07 | 1.130.14 | 0.68 | |
pMTOR (Ser 2481) | 1.000.16 | 0.960.07 | 0.610.08 | 0.03 | * |
Insulin signaling protein expression
There was increased expression of pro-insulin signaling protein PI3K, decreased expression of pro-insulin signaling proteins AKT and AMPK, and increased expression of insulin insensitivity marker GSK3 in the HCVOD group compared to HCC (Table 2). There was decreased expression of pro-insulin signaling proteins AKT, pAKT (Thr 308), pAKT (Ser 473) and MTOR, and increased expression of insulin insensitivity marker GSK3 in the HCW group compared to HCW (Table 2).
GLUT1 and GLUT4 tissue staining in ischemic myocardium
There was a significant increase in membrane-bound GLUT1 staining in the HCVOD and HCW groups compared to HCC (2586.0 309.0, 2535.4 423.6, 1275 127.6 integrated optical density respectively p = 0.01). Also, there was an increase in membrane-bound GLUT4 staining in the HCVOD and HCW groups compared to HCC, but only the HCW group reached significance compared to HCC (106922.9 8172.6, 121662.1 11782.4, 77709.3 3314.6 integrated optical density respectively p = 0.003) (Figure 1).
Oil red O and PAS staining in ischemic myocardium
There was increased intramyocardial lipid deposition in the HCC and HCW groups compared to the HCVOD group (Figure 2) on oil red-O staining. There was very little intramyocardial lipid deposition in the HCVOD group. PAS staining for intramyocardial glycogen storage was homogenous in distribution, and similar in intensity in the HCC, HCVOD and HCW groups (Figure 2).
Figure 1
GLUT1 and GLUT4 Immunohistochemistry in chronically ischemic myocardium. GLUT1 (red) and GLUT4 (green) staining. GLUT1: glucose transporter type 1. GLUT4: glucose transporter type 4. HCC: High Cholesterol Control. HCW: High Cholesterol + Wine. HCVOD: High Cholesterol + Vodka.
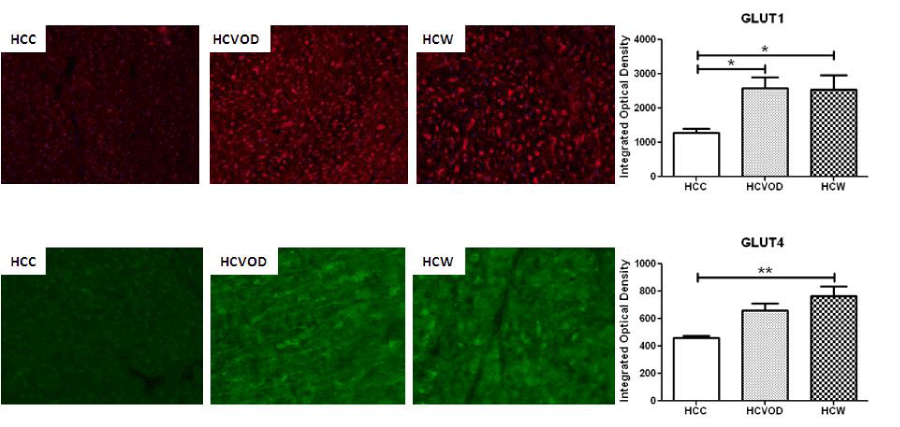
Figure 2
Oil Red-O and Periodic Acid Schiff (PAS) staining of the chronically ischemic myocardium. Arrows indicate positive staining (red) for intramyocardial lipid deposits. HCC: High Cholesterol Control. HCW: High Cholesterol + Wine. HCVOD: High Cholesterol + Vodka.
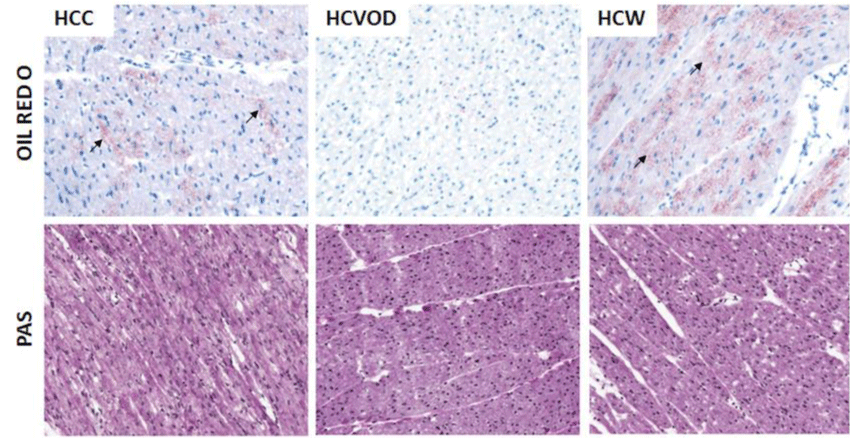
Discussion
Insulin signaling plays a critical role in the ischemic myocardium as it inhibits free fatty acid release, which inhibits free fatty acid-mediated reduced contractility, oxidative stress, and endothelial dysfunction [18-20]. Insulin also promotes myocardial glucose uptake and activates pyruvate dehydrogenase and aerobic metabolism [18]. The cardioprotective effects of insulin and insulin signaling translate to improved patient outcomes after coronary artery bypass surgery, and strict normoglycemia with insulin infusion can reduce operative mortality by 50% [21,22]. We previously reported that wine and vodka supplementation improved myocardial perfusion and reduced myocardial scarring, but only wine supplementation increased perfusion during ventricular pacing, improved segmental shortening and microvessel function and reduced oxidative stress [16]. Given alcohol's known dose-dependent effects on insulin sensitivity, we sought to elucidate the effects of moderate alcohol consumption in a clinically relevant swine model of chronic myocardial ischemia and metabolic syndrome. We previously reported that animals in this study develop components of early metabolic syndrome including obesity, hyperlipidemia, and insulin resistance [16,17]. We also reported that there was aberrant insulin signaling in the liver and skeletal muscle of animals supplemented with resveratrol-containing alcohol, red wine, and resveratrol-free alcohol, vodka [17]. However the effects of alcohol in the chronically ischemic myocardium are unclear. In the present study, we report that at baseline, before animals were supplemented with alcohol, all animals had similar glucose tolerance. After 7 weeks of alcohol supplementation, all animals had similar baseline blood glucose, but animals supplemented with vodka and wine had elevated blood glucose at 30 and 60 minutes after dextrose challenge. This result is expected as we previously reported that these animals were insulin resistant after alcohol supplementation.
We also reported that there was an increase in oil red-O staining in the livers of the HCW and HCC animals, and a lack of intrahepatic lipid deposition in the HCVOD animals [17]. In the present study, we found that cardiac steatosis mirrored the same trend as hepatic steatosis: there were increased intramyocardial lipids in the HCC and HCW animals, and a marked paucity of intramyocardial lipid deposition in the HCVOD group. Hepatic steatosis can occur as a result of chronic ethanol consumption, which impairs lipid metabolism, causes inflammation, oxidative stress and increases lipid accumulation. While steatosis primarily occurs in the liver, it can also occur in the heart, kidney, and skeletal muscle. Cardiac steatosis can also occur as a result of metabolic syndrome [23]. The decreased lipid hepatic and cardiac steatosis may be due to preserved or improved lipid metabolism in the HCVOD group, and further investigation is warranted to determine the mechanism.
In addition to the observed insulin resistance and glucose intolerance, there was also biochemical evidence for aberrant insulin signaling in the HCVOD and HCW animals. The insulin signaling pathway is initiated when insulin binds the insulin receptor resulting in tyrosine phosphorylation of IRS, which in turn activates PI3K [24] (Figure 3). PI3K activates AKT, a serine kinase, which is a key component of the insulin signaling cascade that activates multiple pathways and translocation events. AKT inhibits GSK3, which is an inhibitor of glycogen synthase. Therefore, AKT effectively activates glycogen synthase, which improves glucose utilization and glycogen synthesis. In a well-fed state, AMPK and MTOR are activated, which further propagate the insulin signaling pathway.
Although the histologic staining for glycogen stores did not reveal any difference in morphology or quantity of intramyocardial glycogen storage between groups, there were significant differences in insulin signaling. There was aberrant activation of the insulin signaling pathway with decreased expression of AKT (both HCVOD and HCW), pAKT (HCW), AMPK (HCVOD), and pMTOR (HCW). There was also increased expression of GSK3, a marker of insulin insensitivity in both HCVOD and HCW. There was an increase in PI3K expression in the HCVOD, but the overall trend matches the serologic data suggesting that insulin insensitivity and glucose intolerance in the animals supplemented with vodka and wine.
The down-stream target of insulin signaling is the glucose transporter. In the heart, GLUT1 and GLUT4 are primarily responsible for myocardial glucose transport, with the former mediating basal glucose transport, and the later mediating inducible glucose transport in response to insulin or increased contractility [25]. AKT triggers the translocation of intracellular GLUT4 vesicles to the plasma membrane, where it facilitates the movement of glucose into the cell, improves serum glucose utilization, and reduces serum glucose levels. GLUT1 can also translocate to the plasma membrane in response to insulin or contraction similar to GLUT4 [26]. In conditions of ischemia, where there is an increase in AMP to ATP ratio, AMPK is activated, which unmasks existing GLUT1 transporters at the plasma membrane and substantially increases GLUT1 mediated glucose transport [27].
Interestingly, despite the insulin insensitivity, glucose intolerance, and aberrant insulin signaling, there was increased myocardial expression of GLUT1 and GLUT4 at the plasma membrane on immunohistochemistry in the animals supplemented with alcohol. Studies have shown that low-moderate alcohol consumption improves insulin sensitivity by up-regulating AKT, which promotes insulin signaling, inhibits gluconeogenesis, increases GLUT4 expression, and by up-regulating insulin sensitizers such as adiponectin, and increasing pancreatic insulin secretion [28,29]. However, high alcohol consumption worsens insulin sensitivity by impeding insulin binding the insulin receptor, decreasing IRS activation, and decreasing expression of down-stream targets PI3K, AKT, and GLUT4 [29,30]. Perhaps the dose is given in this study, 45g ethanol daily, represents the "tipping point" at which the anti-diabetic effects of low-moderate alcohol shifts the balance towards pro-diabetic effects of chronic high levels of alcohol in the setting of metabolic syndrome.
Figure 3
Schematic of the insulin signaling pathway. IRS: insulin receptor substrate, PI3K: phosphoinositide 3-kinase, AKT: protein kinase B, GSK-3: glucose synthase kinase-3, GS: glucose synthase, MTOR: mammalian target of rapamycin, AMPK: AMP-activated protein kinase, GLUT1: aglucose transporter type 1, GLUT4: glucose transporter type 4.
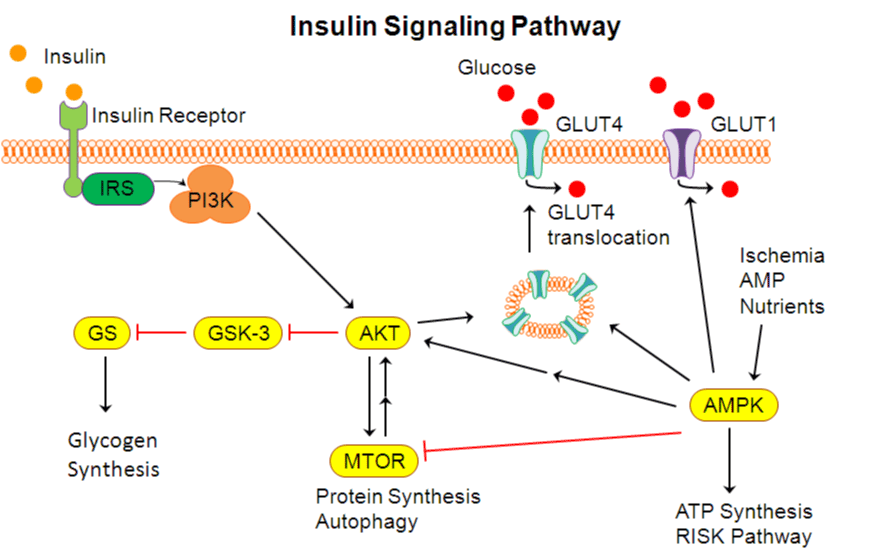
Conclusions
In this clinically relevant model of alcohol supplementation in a swine model of metabolic syndrome and chronic myocardial ischemia, we found that alcohol supplementation decreased glucose tolerance, and altered the insulin signaling pathway by up-regulating pro-insulin signaling proteins PI3K, GLUT1 and GLUT4, down-regulating insulin signaling activators AKT, pAKT, AMPK, pMTOR, and increasing expression of insulin insensitivity marker GSK3. The mixed signals may result in the observed insulin insensitivity. Moderate alcohol consumption seems to have a complex and negative effect on insulin signaling in ischemic myocardium. We previously reported that 45g of ethanol daily promotes insulin signaling in ischemic myocardium in the absence of metabolic syndrome [15]. It is possible that the cardioprotective and anti-diabetic effects of low-moderate alcohol consumption are attenuated with metabolic syndrome. At this time, the mechanism by which alcohol mediates its cardioprotective and anti-diabetic effects is not completely understood. Further investigation on the dose dependent effect of alcohol on myocardial insulin signaling is warranted in future studies.
Limitations
There are several limitations of the current study. Firstly, the study was limited to 7 weeks of alcohol supplementation and animals were all harvested at one-time point. It is possible alcohol modulates insulin signaling differently at varying time points. Secondly, only one dose of alcohol was given in this study. It is well established that alcohol has a dose dependent effect on coronary artery disease and insulin signaling. It would have been useful to repeat this experiment using multiple doses of alcohol to determine the dose response of alcohol on insulin signaling. Lastly, although this is a well-established and validated porcine model of chronic myocardial ischemia, animal models do not completely replicate human disease.
Funding acknowledgement
Funding for this research was provided by the National Heart, Lung, and Blood Institute (R01HL46716, R01HL128831 Dr. Sellke), NIH Training grant 5T32-HL076134 (Dr. Lassaletta), NIH Training grant 5T32-HL094300-03, Drs Chu, Sabe, and Elmadhun).
References
- Wild S, Roglic G, Green A, Sicree R, King H. Global prevalence of diabetes: Estimates for the year 2000 and projections for 2030. Diabetes Care. 2004; 27: 1047-1053.
- Ford ES, Giles WH, Mokdad AH. Increasing prevalence of the metabolic syndrome among u.s. Adults. Diabetes Care. 2004; 27: 2444-2449.
- Mensah GA, Mokdad AH, Ford E, Narayan KM, Giles WH, Vinicor F, et al. Obesity, metabolic syndrome, and type 2 diabetes: Emerging epidemics and their cardiovascular implications. Cardiol Clin. 2004; 22: 485-504.
- Haffner SM, Lehto S, Ronnemaa T, Pyorala K, Laakso M. Mortality from coronary heart disease in subjects with type 2 diabetes and in nondiabetic subjects with and without prior myocardial infarction. N Engl J Med. 1998; 339: 229-234.
- National Cholesterol Education Program Expert Panel on Detection E, Treatment of High Blood Cholesterol in A. Third report of the national cholesterol education program (ncep) expert panel on detection, evaluation, and treatment of high blood cholesterol in adults (adult treatment panel iii) final report. Circulation. 2002; 106: 3143-3421.
- Smiley DD, Umpierrez GE. Perioperative glucose control in the diabetic or nondiabetic patient. South Med J. 2006; 99: 580-589.
- Wannamethee SG, Camargo CA, Jr, Manson JE, Willett WC, Rimm EB. Alcohol drinking patterns and risk of type 2 diabetes mellitus among younger women. Arch Intern Med. 2003; 163: 1329-1336.
- Beulens JW, Stolk RP, van der Schouw YT, Grobbee DE, Hendriks HF, Bots ML. Alcohol consumption and risk of type 2 diabetes among older women. Diabetes Care. 2005; 28: 2933-2938.
- Koppes LL, Dekker JM, Hendriks HF, Bouter LM, Heine RJ. Moderate alcohol consumption lowers the risk of type 2 diabetes: A meta-analysis of prospective observational studies. Diabetes Care. 2005; 28: 719-725.
- Hodge AM, English DR, O'Dea K, Giles GG. Alcohol intake, consumption pattern and beverage type, and the risk of type 2 diabetes. Diabet Med. 2006; 23: 690-697.
- Belleville J. The french paradox: Possible involvement of ethanol in the protective effect against cardiovascular diseases. Nutrition. 2002; 18: 173-177.
- Burgess TA, Robich MP, Chu LM, Bianchi C, Sellke FW. Improving glucose metabolism with resveratrol in a swine model of metabolic syndrome through alteration of signaling pathways in the liver and skeletal muscle. Arch Surg. 2011; 146: 556-564.
- Elmadhun NY, Sabe AA, Robich MP, Chu LM, Lassaletta AD, Sellke FW. The pig as a valuable model for testing the effect of resveratrol to prevent cardiovascular disease. Ann N Y Acad Sci. 2013; 1290: 130-135.
- Sabe AA, Elmadhun NY, Robich MP, Dalal RS, Sellke FW. Does resveratrol improve insulin signaling in chronically ischemic myocardium? J Surg Res. 2013; 183: 531-536.
- Elmadhun NY, Lassaletta AD, Burgess T, Sabe AA, Sellke FW. Alcohol consumption improves insulin signaling in the myocardium. Surgery. 2013; 154: 320-327.
- Chu LM, Lassaletta AD, Robich MP, Liu Y, Burgess T, Laham RJ, et al. Effects of red wine and vodka on collateral-dependent perfusion and cardiovascular function in hypercholesterolemic swine. Circulation. 2012; 126: S65-72.
- Elmadhun NY, Lassaletta AD, Chu LM, Bianchi C, Sellke FW. Vodka and wine consumption in a swine model of metabolic syndrome alters insulin signaling pathways in the liver and skeletal muscle. Surgery. 2012; 152: 414-422.
- Lazar HL. Glycemic control during coronary artery bypass graft surgery. ISRN Cardiology. 2012; 2012: 292490.
- Liu Q, Docherty JC, Rendell JC, Clanachan AS, Lopaschuk GD. High levels of fatty acids delay the recovery of intracellular ph and cardiac efficiency in post-ischemic hearts by inhibiting glucose oxidation. J Am Coll Cardiol. 2002; 39: 718-725.
- Langouche L, Vanhorebeek I, Vlasselaers D, Vander Perre S, Wouters PJ, Skogstrand K, et al. Intensive insulin therapy protects the endothelium of critically ill patients. J Clin Invest. 2005; 115: 2277-2286.
- Lazar HL, Philippides G, Fitzgerald C, Lancaster D, Shemin RJ, Apstein C. Glucose-insulin-potassium solutions enhance recovery after urgent coronary artery bypass grafting. J Thorac Cardiovasc Surg. 1997; 113: 354-360.
- Furnary AP, Gao G, Grunkemeier GL, Wu Y, Zerr KJ, et al. Continuous insulin infusion reduces mortality in patients with diabetes undergoing coronary artery bypass grafting. J Thorac Cardiovasc Surg. 2003; 125: 1007-1021.
- Nyman K, Graner M, Pentikainen MO, Lundbom J, Hakkarainen A, Siren R, et al. Cardiac steatosis and left ventricular function in men with metabolic syndrome. J Cardiovasc Magn Reson. 2013; 15: 103.
- Bertrand L, Horman S, Beauloye C, Vanoverschelde JL. Insulin signalling in the heart. Cardiovasc Res. 2008; 79: 238-248.
- Schwenk RW, Luiken JJ, Bonen A, Glatz JF. Regulation of sarcolemmal glucose and fatty acid transporters in cardiac disease. Cardiovasc Res. 2008; 79: 249-258.
- Wheeler TJ, Fell RD, Hauck MA. Translocation of two glucose transporters in heart: Effects of rotenone, uncouplers, workload, palmitate, insulin and anoxia. Biochim Biophys Acta. 1994; 1196: 191-200.
- Abbud W, Habinowski S, Zhang JZ, Kendrew J, Elkairi FS, Kemp BE, et al. Stimulation of amp-activated protein kinase (ampk) is associated with enhancement of glut1-mediated glucose transport. Arch Biochem Biophys. 2000; 380: 347-352.
- Funaki M, Randhawa P, Janmey PA. Separation of insulin signaling into distinct glut4 translocation and activation steps. Mol Cell Biol. 2004; 24: 7567-7577.
- Guerre-Millo M, Gervois P, Raspe E, Madsen L, Poulain P, Derudas B, et al. Peroxisome proliferator-activated receptor alpha activators improve insulin sensitivity and reduce adiposity. J Biol Chem. 2000; 275: 16638-16642.
- Szkudelski T, Szkudelska K. Anti-diabetic effects of resveratrol. Ann N Y Acad Sci. 2011; 1215: 34-39.
Authors submit all Proposals and manuscripts via Electronic Form!