Visith Sitprija1* and Siravit Sitprija2
1Queen Saovabha Memorial Institute, Thailand
2Department of Biology, Faculty of Science, Mahidol University, Thailand
*Address for Correspondence: Visith Sitprija, Queen Saovabha Memorial Institute, 1871 Rama 4 Road, Bangkok 10330, Thailand
Dates: Submitted: 30 September 2016; Approved: 04 December 2016; Published: 06 December 2016
Citation this article: Sitprija V, Sitprija S. Renal Injury Induced by Marine Toxins : Role of Ion Channels. Int J Nephrol Ther. 2016;2(1): 001-006
Copyright: © 2016 Sitprija V, et al. This is an open access article distributed under the Creative Commons Attribution License, which permits unrestricted use, distribution, and reproduction in any medium, provided the original work is properly cited.
Keywords: Renal injury; Domoic acid; Palytoxin; Maitotoxin; Cnidarian toxins; Ion channels; Pore formation
Abstract
Renal injury by marine toxins is not common. Domoic acid, palytoxin and maitotoxin from planktons and cnidarian toxins are few toxins known to cause renal injury. They target ion channels or form pores on the cell membrane, which increase cytosolic Na+ and Ca2+ and cause cellular edema leading to cell death. The mechanism differs from what observed in the other causes of renal injury by animal toxins. This is a model of renal injury by disruption of cell volume regulation.
Introduction
Injury by animal toxins is common in the tropics. As a vascularized organ the kidney is an important target of toxins. Snake and arthropod venoms with destructive enzymes are well known causes of renal injury and have received much attention [1]. Inflammatory reaction with hemodynamic changes plays important roles in renal injury. Marine toxins are an area of interest with few data on renal injury. Toxins from planktons and fauna are known to have effects on ion channels, and often cause neurological, cardiovascular and gastrointestinal symptoms [2]. Some toxins, targeting both excitable and non-excitable cells, can cause renal injury [3-5]. In cnidarian envenomation, renal injury is known in jellyfish stings [6,7] with a recent report of Acute Kidney Injury (AKI) due to sea anemone [8]. Renal injurious effects of both marine and cnidarian toxins, either by direct or indirect, are due to increased cytosolic sodium and calcium and cell swelling through ion channels transport. Clinical data are limited. Due to environmental changes, spreading of marine toxins are becoming more prominent along the coast line and can accumulate in fish, clams and mussels. Consumption of toxin contaminated sea food can cause adverse effects on health. In keeping with global environmental changes, renal injury by marine toxins deserves more clinical and physiological attention.
Among marine toxins domoic acid, palytoxin, maitotoxin and cnidarian toxins are known to be nephrotoxic or potentially nephrotoxic.
Domoic Acid (DA)
DA is structurally related to Kainic Acid (KA) and glutamic acid, sharing glutamate receptors. DA, produced by plankton Pseudo-nitzschia and red alga Chandria armata, is a potent neurotoxin with effects on gastrointestinal, cardiovascular and renal systems [9]. Toxin contaminated shellfish and crustaceans in the food chain consumed by man can cause toxicity. The blue mussel (Mytilus edulis) is the most common vector. Clinically, DA is the cause of amnesic shellfish poisoning. Gastrointestinal symptoms, occurring with 24h, include nausea, vomiting, diarrhea and abdominal cramp. Cardiac arrhythmias and unstable blood pressure can be observed. Neurological symptoms are manifested as seizure and coma with memory loss within 48h [10,11].
Both DA and KA are excitotoxic by activation of ionotropic glutamate receptors (iGluRs) including α Amino-3-hydroxy-5-Methyl-4-isozazole Propionic Acid (AMPA), KA and N-Methyl-D-Aspartate (NMDA) receptor subtypes which increase cation transport of Na+, K+ and Ca2+ through nonselective cation channels. Ca2+ influx predominates for NMDA receptor and enhances Ca2+ efflux from endoplasmic reticulum. DA also decreases cAMP through inhibition of Ca2+ and calmodulin stimulated adenylate cyclase [12]. Decreased PKA, resulted from decreased cAMP, inhibits Ca2+ ATPase further increasing cytosolic Ca2+. Increased cytosolic Ca2+ causes excitation and ultimately leads to cell death through ROS, PLA2 and caspase-3 pathway [13]. In addition, increased cytosolic Ca2+ causes actin depolymerization and enhances Na+ influx by up-regulation of NHE and NKCC. In mice, DA preferentially accumulates in the kidney especially in the proximal tubules and causes tubular and endothelial damage [14]. Both uNGAL and uKIM-1 are elevated. Acute phase genes c-fos and junb are induced. Pretreatment with kynurenic acid, an excitatory aminoacid inhibitor, attenuates the response. Of interest, the dose of DA in causing renal injury is much less than that causing neuron injury. Renal injury by DA in man has not been described.
Palytoxin
Palytoxin is chemically a fatty alcohol produced by dinoflagellate Ostreopsis ovate. Palytoxin poisoning is caused by consumption of coral fish such as trigger fish, parrot fish, clupeoid fish, crab, sardine fed on dinoflagellates. Toxicity is due to inhibition of Na+-K+ ATPase at the binding site of ouabain [15]. Renal epithelial cells are also toxin target [16]. The cell becomes depolarized with opening of Na+ and Ca2+ channels. Na+, Ca2+ and water influx causes cell swelling. Ca2+ influx is not inhibited by verapamil or nefedipine, and it is believed that Ca2+ influx is through SKF-96365 or Ni2+ sensitive channel (Cav3.x) [17]. The toxin also forms pores on the cell membrane further enhancing Ca2+ and Na+ influx. Palytoxin increases intracellular acidification by increasing mitochondrial Ca2+ uptake with displacement of H+ ion [18]. Increased cytosolic Na+ also decreases NHE activity and decreases H+ ion efflux. Increased cytosolic Ca2+ in the vascular smooth muscle cells stimulates myosin kinase which causes vascular contraction and hypertension. Massive Ca2+ influx can cause cell death. The symptoms of palytoxin poisoning include nausea, vomiting, abdominal cramp, diarrhea, and muscle pains [5]. Bronchial constriction, respiratory distress, hypertension, bradycardia, rhabdomyolysis, hemolysis, hyperkalemia and renal failure are among the severe complications [4,19,20]. A recent study showed palytoxin inhibition of H+-K+ ATPase in the distal colon [21]. This represents the counteracting mechanism to decrease serum K+ by inhibition of K+ absorption in the distal colon. Since palytoxin causes injury of renal cells, erythrocytes and muscle cells, acute renal failure can be due to combination of direct renal injury and indirect injury by myoglobinuria or hemoglobinuria.
Maitotoxin
Maitotoxin consists of large fatty acid chains produced by dinoflagellate Gambierdiscus toxicus. Toxicity is induced by consumption of fish, mainly coral reef fish, fed on these dinoflagelletes. Baracuda, snapper, grouper, jacks and morey eel are among those fish with possible high toxin load. Clinical symptoms include numbness of perioral area and extremities, myalgia, headache, itching, hemolysis, hypertension, headache, blurred vision, arrhythmias and paralysis [3]. The toxin activates both nonselective cation channels (TRPM2) and epithelial Ca2+ channels (TRPV5) and forms pores on the cell membrane. Maitotoxin converts the plasmolemmal Ca2+ ATPase to Ca2+ permeable nonselective cation channel [22]. Increased Ca2+, Na+ and water influx results in increased cytosolic Ca2+ and Na+ and cell swelling. MDCK cells and HEK cells are also targeted of renal Ca2+ influx through TRPV5 [23-25]. Although large pore formation by maitotoxin resembles P2Z/P2X7 receptor induced pores, it is P2Z/P2X7 and ATP independent. Sequentially maitotoxin increases Ca2+ influx through nonselective cation channels, opens cytolytic/oncotic pores and causes cell death. This is reflected by increased uptake of vital dyes such as ethidium and propidium followed by the release of lactate dehydrogenase [26]. By Ca2+ influx, maitotoxin increases secretion of norepinephrine and dopamine inhibited by verapamil, indicating that Ca2+ influx is through L-type Ca2+ channels (Cav1.1-1.4) [27]. Activation of inflammatory reactions through cytokine secretion is another insult in addition to increased cytosolic Ca2+ that leads to cell injury [28]. Despite physiological evidence of renal involvement clinical and pathological data are not available. Clinically, only oliguria was described without laboratory data [29].
Cnidarian toxins
Important toxic components of cnidarians consist of pore forming toxins, membrane attack complex perforin, toxins acting on voltage gated K+ and Na+ channel, Acid Sensing Ion Channel (ASIC), TRPV1, small cysteine rich peptides, histamine, hyaluronidase, fibrinolysis, kinins and serotonin [30]. The severity of envenomation depends on several factors including age, size of the patient, surface area of contact, duration of contact and the cnidarian species. All cnidarians are potentially nephrotoxic. Clinical reports are from jellyfish and some Anemone envenoming.
Pore forming toxins
Pore forming toxins are present in cnidarians of Classes Anthozoa, Hydrozoa, Cubozoa and Scyphozoa [31]. They are important in causing injury to the preys and predators including humans. Toxins bind phosphocholine lipid and sphingomyelin and form pores on cell membrane of all cells including cardiac muscle cells, erythrocytes and kidney cells [32]. Pore formation results in Ca2+, and Na+ influx and K+ efflux. Ion gradient between intracellular and extracellular fluid is dissipated. Internal and external ions equilibrate. Cellular influx of Na+ and water causes cell swelling. Increased cytosolic Ca2+ by Ca2+ influx activates K+ channel (Kca) further enhancing K+ efflux. High cytosolic Ca2+ also enhances Ca2+ efflux from endoplasmic reticulum and sarcoplasmic reticulum through activation of ryanodine receptor. Release of catecholamine from sympathetic neurons and acetylcholine from parasympathetic neurons induced by increased cytosolic Ca2+ causes unstable blood pressure and gastrointestinal symptoms. Both hypotension and hypertension can be observed, but usually hypertension predominates. Box jellyfish envenoming causes hemolysis, coronary vasoconstriction which can result in cardiac arrest. Through pore formation and ion channels, injury by cnidarian toxins bears some resemblance to that of spider latrotoxin, bee melittin and some bacterial toxins. Loss of intermediates of metabolism, macromolecules and continuing rise in cytosolic Ca2+ can cause cell death. Renal pathological changes include tubular degeneration, vascular congestion, hemorrhage and shrinkage of glomerular tuft [33,34].
Clinical symptoms of cnidarian injury are mainly due to pore forming toxins. Local symptoms of cnidarian envenoming due to enzymes includes pain, erythema, edema and vascular lesion. Systemic symptoms vary from mild to severe consisting of weakness, nausea, vomiting, diarrhea, muscle spasm, paresthesia, hypertension, pulmonary edema, respiratory arrest, cardiac arrhythmias, cardiac arrest and acute renal failure. Intravascular hemolysis and rhabdomyolysis can be observed. Injury by cubozoans including box jellyfish (Chironex fleckeri), four-handed box jellyfish (Chiropsalmus quadrigatus) and Irukandji jellyfish (Carukia barnesi) is most severe and can cause cardiovascular death quickly even before diagnosis of AKI can be made [35-38]. Portuguese man-of-war (Physalia physalis) in class Hydrozoa causes hypertension, intravascular hemolysis and renal failure [7,39]. Chirodropid, a large box-shaped jellyfish, also causes hemoglobinuria and renal failure [40]. Minimal change disease with heavy proteinuria has been reported following contact with fire coral (Millepora species) in class Hydrozoa [41]. Envenomings by sea nettle (Chrysaora), pelagia jellyfish (Pelagia) and others in Scyphozoa class are less severe [6,42,43].
Equinatoxin from common sea anemone (Actinia equina) in Class Anthozoa is most toxic and can cause death by cardiac arrest form coronary vasoconstriction and hyperkalemia in mice. Massive Ca2+ and Na+ influx into the vascular smooth muscle cells or coronary arteries can cause intense vasoconstriction [44,45]. Hyperkalemia due to K+ outflux from the cell especially intravascular hemolysis further contributes to cardiac arrest. Tezosentan, an inhibitor of equinatoxin II and endothelin I, has potential role in equinatoxin envenoming [46]. Garcia et al. described a patient stung by sea anemone (Condylactis species) developing severe hepatic and renal failure [47].
Membrane Attack Complex-Perforin (MACP)
MACP proteins are produced by night anemone (Phyllodiscus semoni) and Okinawan anemone (Actinaria villosa) of Anthozoa class. The toxin is structurally related to cholesterol dependent cytolysin produced by T cells and killer cells which forms pores on the target membrane. MACP is required for membrane insertion of C8a and C9 [48]. Tissue injury is induced by complement activation and transmembrane pore formation. This toxin heavily activates complement causing vascular, glomerular and tubular injury and creates a transmembrane pore in target cells leading to apoptosis. In addition to severe dermatitis due to toxin exposure the victim develops severe AKI with hemolytic uremic syndrome [8].
Enzymes
Phospholipase A2 (PLA2) and metalloprotease contribute to inflammatory reactions and tissue injury. Both enzymes can induce hemodynamic alteration and renal ischemia that can also lead to renal injury. PLA2 is present in class Anthozoa, Hydrozoa, Scyphozoa and Cubozoa. Metalloproteases are detectable in cannonball jellyfish (Stomolophus meleagris), box jellyfish (Chironex fleckeri) and starlet sea anemone (Nematostella vectensis) [30]. Nephrotoxicity of cnidarian toxin through rhabdomyolysis and intravascular hemolysis is another possibility. Clinical symptoms of injury by marine and cnidarian toxins are summarized in (Table 1).
Physiological consideration
As shown on (Figure 1), in all instances the net result is increased cytosolic Na+ and Ca2+, cellular edema and cell death. Pore formation on cell membrane also causes hyperkalemia. Normally, cell volume is regulated by cytoskeleton sensing through integrin and F-actin interaction which controls ion transporters [49,50]. For cell volume decrease in the situation of cellular edema, Na+ influx is inhibited along with stimulation of Na+-K+ ATPase and opening of K+ channels. Cell volume regulation to decrease cell volume fails to function in the toxin setting. In cnidarian injury, Na+ channels are activated, and pores on the membrane allow free transport of Na+, K+ and Ca2+ [51]. In palytoxin poisoning Na+-K+ ATPase is inhibited. Down gradient Na+ and Ca2+ influxes are further enhanced through pores. Normally cellular response to increased cytosolic Ca2+ includes upregulation of Ca2+ ATPase either PMCA or SERCA and by Ca2+ efflux through increased Na2+-Ca2+ Exchange (NCX). In DA poisoning Ca2+ ATPase is inhibited by decreased cAMP and PKA [12]. In palytoxin poisoning NCX is inhibited by increased intracellular Na+. In maitotoxin toxicity Ca2+ ATPase is converted to non selective cation channels [22]. Therefore, cytosolic Ca2+ remains high in DA, palytoxin, maitotoxin and cnidarian toxin injury. Increased cytosolic Ca triggers activation of several enzyme activities including phospholipase, PKC, proteases, protein phosphatases, calmodulin activated NO synthase, free radicals caspase - 3 pathway and actin depolymerization. PLA2 activates generation of arachidonic acid. Cysteine transport is inhibited, which decreases sulphydryls with generation of oxygen radicals contributing to apoptosis and necrosis. Pore formation on the membrane disrupts cellular ion homeostasis. In severe case, disruption can be beyond cell volume regulation with loss of macromolecules, amino acids and protein resulting in cell death. It has been suggested that membrane leakage could be ameliorated by divalent cations such as Zn2+, Mg2+ and Ni2+ [52,53]. Ni2+ closes T-type Ca2+ channels. Inhibition of NKCC1 by bumetanide may decrease cytosolic Na+ and decrease cytosolic Ca2+ by NCX. The mechanism of pore forming remains unclear. Pore forming effect of maitotoxin is believed to be related to high Ca2+ influx, independent of purinergic receptor activation and ATP [26]. This may be also true for palytoxin effect. It is puzzling that DA does not cause pore forming.
High cytosolic Ca2+ stimulates increased synthesis of catecholamines in sympathetic neurons causing hypertension and cardiac arrhythmias. Increased acetylcholine synthesis in parasympathetic neurons causes gastrointestinal symptoms including nausea, vomiting and diarrhea which are commonly observed. Although blood pressure may be unstable, hypertension is common, and may not respond to L-type Ca2+ and Na+ blockers. Severe hypertension and coronary vasoconstriction are observed in box jellyfish causing rapid death. Erythrocytes, muscle cells, vascular smooth muscle and renal tubular cells are susceptible to increased cytosolic Ca2+ and cellular edema at various degrees. Erythrocytes are more sensitive. Therefore hemolysis is common. In a study of Pelagia noctiluca jellyfish venom, oxidative stress due to toxin, reflected by decreased GSH, decreases SO4 uptake in erythrocyte band 3 protein and increases Cl-depedent K+ efflux. With this compensatory mechanism erythrocyte volume is decreased [54]. Hyperkalemia can therefore be observed before lysis of erythrocytes. Zinc gluconate inhibits K+ efflux and could be useful in cubozoan envenomation [55]. MgSO4 has been used in cubozoan hypertension. Hemolysis and rhabdomyolysis are common findings with wide variability. Cnidarian enzymes can also induce inflammatory reaction. PLA2, metalloprotease, inflammatory cytokines, complement activation, hemolysis and rhabdomyolysis play additional role in tissue and renal injury.
Table 1
Table 1: Clinical symptoms and renal injury by marine toxins. | |||
Clinical data | Renal involvement | Reference | |
Domoic acid (Pseudo nitzschia) |
non | AKI in mice (Intraperitoneal) | [14] |
Palytoxin (Ostreopsis ovata) |
Gastrointestinal symptoms Hypertension Bronchial constriction Muscle pain Rhabdomyolysis |
AKI (ingestion) | [4,5,19] |
Maitotoxin (Gambierdiscus toxicus) |
Numbness Paralysis Arrhythmia Hypertension Myalgia Headache |
Oliguria (ingestion) | [29] |
Cnidarian toxin
-Portuguese man-of-war (Physalia physalis) |
Pain Erythema Edema Weakness, Gastrointestinal symptoms Hypertension |
AKI (contact) | [7,39] |
-Night sea anemone (Phyllodiscus semoni) |
Dermatitis Pain Pulmonary edema Hemolytic uremic syndrome |
AKI Thrombotic microangiopathy (sting) |
[8] |
- Sea anemone (Anthopleura asiatica) |
Non | AKI in mice (intraperitoneal) | [33] |
- Sea anemone (Condylactis species) |
Hemorrhagic vesicle Hepatitis |
AKI (sting) |
[47] |
- Fire coral (Millepora species) |
Pain Blister Edema |
Minimal change disease | [41] |
- Chirodropid box jellyfish (Chiropsella rudloei) |
Dermatitis Hemoglobinuria |
AKI (sting) | [40] |
Figure 1
Showing mechanism of cell death by marine and cnidarians toxins. TRPM2 : Calcium permeable non specific cation channel, TRPV5 : Epithelial calcium channel.
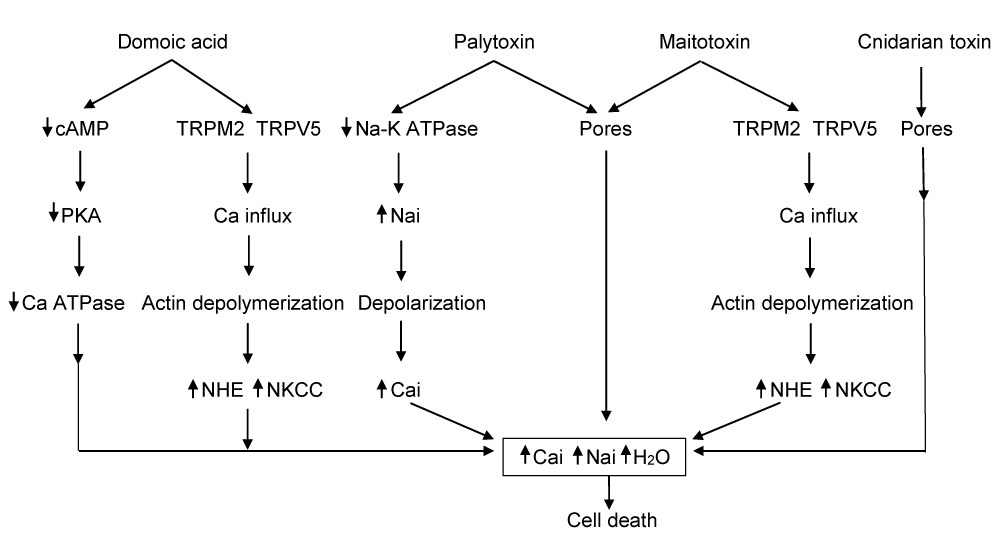
Conclusion
Renal injury by animal toxins is in general, ischemic in nature induced by inflammatory cytokines and vasoactive mediators. Interestingly, renal injury by marine toxins comprising domoic acid, palytoxin, maitotoxin and cnidarian toxins is induced by ion transport through ion channels or pore formation which result in increased cytosolic Na+ and Ca2+ and cell swelling. Cell volume regulation fails to function because of toxin effects. Increased cytosolic Na+ and Ca2+ and loss of macromolecules and nutrients by pore formation cause cell death. Hemolysis and rhabdomyolysis due to erythrocyte and myocyte injury further contribute indirectly to renal tubular cell death in palytoxin, maitotoxin and cnidarians injury. This is another model of renal injury by animal toxins through ion channels.
References
- Sitprija V, Sitprija S. Renal effects and injury induced by animal toxins. Toxicon. 2012; 60: 943-953.
- Cusick K.D, Sayler G.S. An overview on the marine neurotoxin, saxitoxin : genetics, molecular targets, methods of detection and ecological function. Mar Drugs. 2013; 11: 991-1018.
- Lu X.Z, Deckey R, Jiao G.L, Ren H.F, Li M. Caribbean maitotoxin elevates [Ca2+]i and activates non-selective cation channels in HIT-T15 cells. World J Diabetes. 2013; 4: 70-75.
- Shinzato T, Furusu A, Nishino T, Abe K, Kanda T, Maeda T, Kohno S. Cowfish (Umisuzume, Lactoria diaphana) poisoning with rhabdomyolysis. Intern Med. 2008; 47: 853-856.
- Wu M.L, Yang C.C, Deng J.F, Wang K.Y. Hyperkalemia, hyperphosphatemia, acute kidney injury and fatal dysrhythmia after consumption of palytoxin-contaminated goldspot herring. Ann Emerg Med. 2014; 64: 633-636.
- Burnett J.W, Goldner R. Effects of Chrysaora quinquecirrha (sea nettle) toxin on the rat cardiovascular system. Proc Soc Exp Biol Med.1969; 132: 353-356.
- Cegolon L, Heymann W.C, Lange J.H, Mastrangelo G. Jellyfish stings and their management : A review : Mar Drugs. 2013; 11: 523-550.
- Spielman F.J, Bowe E.A, Watson C.B, Klein E.F. Jr. Acute renal failure as a result of Physalia physalis sting. South Med J. 1982; 75: 1425-1426.
- Mizuno M, Ito Y, Morgan B.P. Exploiting the nephrotoxic effects of venom from the sea anemone, Phyllodiscus semoni, to create a hemolytic uremic syndrome model in rat. Mar Drug. 2012; 10: 1582-1604.
- Mos L. Domoic acid : a fascinating marine toxin. Environ Toxicol Pharmacol. 2001; 9: 79-85.
- Perl T.M, Bedard L, Kosatsky T, Hockin J.C, Todd E.C, Remis R.S. An outbreak of toxic encephalopathy caused by eating mussels contaminated with domoic acid. N Engl J Med. 1990; 322: 1775-1780.
- Pulido O.M. Domoic acid toxicologic pathology : a review. Mars Drug. 2008; 6: 180-219.
- Nijjar M.S, Nijjar S.S. Domoic acid-induced neurodegeneration resulting in memory loss is mediated by CA2+ overload and inhibition of Ca2++ calmodulin-stimulated adenylate cyclase in rat brain (review). Int J Mol Med. 2000; 6: 377-389.
- Gill S.S, Pulido O.M. Glutamate receptors in peripheral tissues : current knowledge, future research, and implications for toxicology. Toxicol Path. 2001; 29: 208-223.
- Funk J.A, Janech M.G, Dillon J.C, Bissler J.J, Siroky B.J, Bell P.D. Characterization of renal toxicity in mice administered the marine biotoxin domoic Acid. J Am Soc Nephrol. 2014; 25: 1187-1197.
- Perez-Gomez A, Novelli A, Fernandez-Sanchez M.T. Na+/K+-ATPase inhibitor palytoxin enhances vulnerability of cultured cerebellar neurons to domoic acid via sodium-dependent mechanisms. J Neurochem. 2010; 114: 28-38.
- B?ttinger H, Habermann E. Palytoxin binds to and inhibits kidney and erythrocyte Na+, K+ ATPase. Arch Path. 1984; 325: 85-87.
- Satoh E, Ishii T, Nishimura M. Palytoxin-induced increase in cytosolic-free Ca(2+) in mouse spleen cells. Eur J Pharmacol. 2003; 465: 9-13.
- Vale-Gonzalez C, Gomez-Limia B, Vieytes M.R, Botana L.M. Effects of the marine phycotoxin palytoxin on neuronal pH in primary cultures of cerebellar granule cells. J Neurosci Res. 2007; 85: 90-98.
- Okano H, Masuoka H, Kamei S, Seko T, Koyabu S, Tsuneoka K, Tamai T, Ueda K, Nakazawa S, Sugawa M, Suzuki H, Watanabe M, Yatani R, Nakano T. Rhabdomyolysis and myocardial damage induced by palytoxin, a toxin of blue humphead parrotfish. Intern Med. 1998; 37: 330-333.
- Taniyama S, Arakawa O, Terada M, Nishio S, Takatani T, Mahmud Y, et al. Ostreopsis sp., a possible origin of palytoxin (PTX) in parrotfish Scarus ovifrons. Toxicon. 2003; 42: 29-33.
- Scheiner-Bobis G, Hubschle T, Diener M. Action of palytoxin on apical H+/K+-ATPase in rat colon. Eur J Biochem. 2002; 269: 3905-3911.
- Sinkins W.G, Estacion M, Prasad V, Goel M, Shull G.E, Kunza D.L, Schilling W.P. Maitotoxin converts the plasmalemmal Ca++ pump into a Ca++ Permeable nonselective cation channel. Am J Physiol Cell Physiol. 2009; 297: C1533-C1545.
- Dietl P, Volkl H. Maitotoxin activates a nonselective cation channel and stimulates Ca2+ entry in MDCK renal epithelial cells. Mol Pharmacol. 1994; 45: 300-305.
- Schilling W.P, Wasylyna T, Dubyak G.R, Humphreys B.D, Sinkins W.G. Maitotoxin and P2Z/P2X(7) purinergic receptor stimulation activate a common cytolytic pore. Am J Physiol. 1999; 277: C766-C776.
- Takahashi M, Ohizumi Y, Yasumoto T. Martotoxin C Ca+ channel activator. Pathogenesis of experimental maitotoxin poisoning. Toxicon. 1989; 27: 979-988.
- Estacion M, Schilling WP. BMC Physiology. 2002; DOI : 10.1186/1472.6793.2.2
- Takahashi M, Tatsumi M, Ohizumi Y. Ca2+ channel activating function of maitotoxin, the most potent marine toxin known, in clonal rat pheochromocytoma cells. J Biol Chem. 1983; 258: 10944-10949.
- Verhoef P.A, Kertesy S.B, Estacion M, Schilling W.P, Dubyak G.R. Maitotoxin induces biphasic interleukin-1beta secretion and membrane blebbing in murine acrophages. Mol Pharmacol. 2004; 66: 909-920.
- Hokama Y, Yoshikawa-Ebesu J.S.M. Ciguatera fish poisoning ; a foodborne disease. J Toxicol. 2001; 20: 85-139.
- Jouiaei M, Yanagihara A.A, Madio B, Nevalainen T.J, Alewood P.F, Fry B.G. Ancient venom systems : a review on cnidaria toxins. Toxins. 2015; 7: 2251-2271.
- Frazao B, Vasconcelos V, Antunes A. Sea Anemone (Cnidaria, Anthozoa, Actiniaria) Toxins : An Overview. Mar Drugs. 2012; 10: 1812-1851.
- Bakrac B, Kladnik A, Macek P, McHaffie G, Werner A, Lakey J.H, et al. A toxin-based probe reveals cytoplasmic exposure of Golgi sphingomyelin. J Biol Chem. 2010; 285: 22186-22195.
- Ravindran V.S, Kannan L, Venkateshvaran K. Biological activity of sea anemone proteins: I. Toxicity and histopathology. Indian J Exp Biol. 2010; 48: 1225-1232.
- Ramkumar S, Arun Sudhagar S, Venkateshvaran K. Bioactivity of venom extracted from the sea anemone Anthopleura asiatica (Cnidaria : Anthozoa) : toxicity and histopathological studies. Int J Fish Aquaculture. 2012; 4: 71-76.
- Bailey P.M, Bakker A.J, Seymour J.E, Wilce J.A. A functional comparison of the venom of three Australian Jellyfish-Chrionex fleckeri, Chiropsalmus sp. and Carybdea xaymacana - on cytolic Ca2+, haemolysis and Artemia sp. Lethality. Toxicon. 2005; 45: 233-242.
- Bengston K, Nichols M.M, Schnadig V, Ellis M.D. Sudden death in a child following jellyfish envenomation by Chiropsalmus quadrumanus. JAMA. 1991; 266: 1404-1406.
- Noguchi K, Sakanashi M, Matsuzaki T, Nakasone J, Sakanashi M, Koyama T, Hamadate N, Sakanashi M. Cardiovascular effects and lethality of venom from nematocysts of the box-jellyfish Chiropsalmus quadrigatus (Habu-kurage) in anaesthetized rats. Toxicon. 2005; 45: 519-526.
- Winkel K.D, Tibballs J, Molenaar P, Lambert G, Coles P, Ross-Smith M, Wiltshire C, Fenner P.J, Gershwin L.A, Hawdon G.M, Wright C.E, Angus J.A. Cardiovascular actions of the venom from the Irukandji (Carukia barnesi) jellyfish : effects in human, rat and guinea-pig tissues in vitro and in pigs in vitro. Clin Exper Pharmacol Physiol. 2005; 32: 777-788.
- Guess H.A, Saviteer P.L, Morris C.R. Hemolysis and acute renal failure following Portuguese Man-of-War sting. Pediatrics. 1982; 70: 979-981.
- Fenner P.J, Lippmann J, Gershwin L.A. Fatal and nonfatal severe jellyfish stings in Thai waters. J Travel Med 17. 2010; 17: 133-138
- Ramesh Prasad G.V, Vincent L, Hamilton R, Lim K. Minimal change disease in association with fire coral (Millepora species) exposure. Am J Kidney Dis. 2006; 47: e15-e16.
- Bruschetta G, Impellizzeri D, Morabito R, Marino A, Ahmad A, Spano N, La Spadec G, Cuzzocrea S, Esposito E. Pelagia nectiluca (Scyphozoa) crude venom injection elicits oxidative stress and inflammatory response in rats. Mar Drugs. 2014; 12: 2182-2204.
- Muhvich K.H, Sengottuvelu S, Manson P.N, Myers R.A, Burnett J.W, Marzella L. Pathophysiology of sea nettle (Chrysaora quinquecirrha), envenomation in a rat model and the effects of hyperbaric oxygen and verapamil treatment. Toxicon. 1991; 29: 857-866.
- Suput D, Frangez R, Bunc M. . Toxicon. 2001; 39: 1421-1427.
- Zorec R, Tester M, Macek P, Mason WT. Cytotoxicity of equinatoxin II from the sea anemone Actinia equina involves ion channel formation and an increase in intracellular calcium activity. J Membrane Biol. 1990; 118: 243-249.
- Drevensek G, Kirbis S, Bunc M, Zitko M, Budihna MV, Suput D. Tezosentan inhibits both equinatoxin II and endotelin-1 induced contractions of isolated porcine coronary artery in a similar way. J Nat Toxins. 2002; 11: 231-244.
- Garcia P.J, Schein R.M, Burnett J.W. Fulminant hepatic failure from a sea anemone sting. Ann Intern Med. 1994; 120: 665-666.
- Rosado C.J, Kondos S, Bull T.E, Kuiper M.J, Law R.H, Buckle A.M, Voskoboinik I, Bird P.I, Trapani J.A, Whisstock J.C, Dunstone M.A. The MACPF/CDC family of pore forming toxins. Cell Microbiol. 2008; 10: 1765-1774.
- Rosado C.J, Kondos S, Bull T.E, Kuiper M.J, Law R.H, Buckle A.M, Voskoboinik I, Bird P.I, Trapani J.A, Whisstock J.C, Dunstone M.A. The MACPF/CDC family of pore forming toxins. Cell Microbiol. 2008; 10: 1765-1774.
- Bashford C.L. Pore-forming toxins. Attack and defence at the cell surface. Cell Biol Mol Lett. 2001; 6: 328-333.
- Mustafa M.R, White E, Hongo K, Othman I, Orchard CH. The mechanism underlying the cardiotoxic effect of the toxin from the jellyfish Chironex fleckeri. Toxicol Appl Pharmacol. 1995; 133: 196-206.
- Morabito R, Marino A, Romano P, Rigano C, La Spada G. Sulphate and chloride-dependent potassium transport in human erythrocytes are affected by crude venom from nematocysts of the jellyfish Pelagia noctiluca. Cell Physiol Biochem. 2013; 32 (Suppl 1): 86-95.
- Yanagihara A.A, Shohet R.V. Cubozoan venom-induced cardiovascular collapse is caused by hyperkalemia and prevented by zinc gluconate in mice. PLoS One. 2012; 7: e51368.
Authors submit all Proposals and manuscripts via Electronic Form!